From US Department of Energy NEUP newsletter August 2024
Group perseveres to develop plasma bubble spectroscopy
Three students at North Carolina State University are working to get advanced molten salt reactors on people’s radar.
The team developed a new technique for molten salt characterization, which they call “plasma bubble spectroscopy”
Molten salt nuclear reactors use molten salt as a coolant instead of water. Using molten salt as a coolant has several advantages including enabling the reactor to operate at near atmospheric pressure even at high temperatures and a large capacity for energy storage. In some designs, nuclear fuel, or uranium, is dissolved in the molten salt and sent into the core of the reactor. One of the main challenges with molten salt is that other elements can mix with it, and there is no way of telling what else may be in the fuel. Professor Alex Bataller, doctoral students Kayla Hahn and Davis Bryars, along with undergraduate researcher Alina Jugan, are using Nuclear Energy University Program funds to address this challenge. Their research has made it possible to quantify how much fuel is in the salt at any given time for salt processing and material accountancy. Impressively, this process uses a probe that can withstand the extreme environment of molten salt.
The Process
The team focused on plasma spectroscopy to measure different elements within a molten salt mixture. Plasma spectroscopy uses optical emission to identify and quantify the elemental and isotopic composition of different materials. To do so, an element is brought to an excited electronic state, where it emits a unique spectral fingerprint that can be detected. In simpler terms, different elements emit different types of light when energized. To energize these elements into excited states, the material must first be transformed into an energetic plasma. To achieve this, the team developed a high voltage plasma discharge, also known as a “glow discharge,” directly inside the molten salt. However, making a plasma inside 700 Celsius molten salt is not without its challenges.
The team had to solve two issues to accomplish the measurement they needed, and in doing so, Bataller and the team developed a new technique for molten salt characterization, which they call “plasma bubble spectroscopy.” First, creating a glow discharge inside a molten salt required a high voltage electrode that can withstand the extreme environment with minimal degradation. Second, the light from the plasma needed to be extracted from the molten salt and into a spectrometer. The students eventually identified a sensor that achieved these goals, but the journey took many turns.
“We started doing a lot of research and making designs to figure out how we would implement this,” said Hahn. “We started with distilled water and saline just to make sure our system worked.”
“We started doing a lot of research and making designs to figure out how we would implement this,” said Hahn. “We started with distilled water and saline just to make sure our system worked.”
“We started doing a lot of research and making designs to figure out how we would implement this,” said Hahn. “We started with distilled water and saline just to make sure our system worked.”directly inside the molten salt. However, making a plasma inside 700 Celsius molten salt is not without its challenges.
The team had to solve two issues to accomplish the measurement they needed, and in doing so, Bataller and the team developed a new technique for molten salt characterization, which they call “plasma bubble spectroscopy.” First, creating a glow discharge inside a molten salt required a high voltage electrode that can withstand the extreme environment with minimal degradation. Second, the light from the plasma needed to be extracted from the molten salt and into a spectrometer. The students eventually identified a sensor that achieved these goals, but the journey took many turns.
“We started doing a lot of research and making designs to figure out how we would implement this,” said Hahn. “We started with distilled water and saline just to make sure our system worked.
The group showed their perseverance, running trial after trial. “We came up with designs for how to submerge an electrode or pair of electrodes,” Hahn said. “We wanted to at least make a bubble in the saline. We went through so many designs.”
The team used a salt solution for testing as well to give them insight as to how the probe might respond in molten salts. The students said it could take hours talking about the iterations they went through, and yet they did not give up when trials failed. They considered the failures vital to their research.
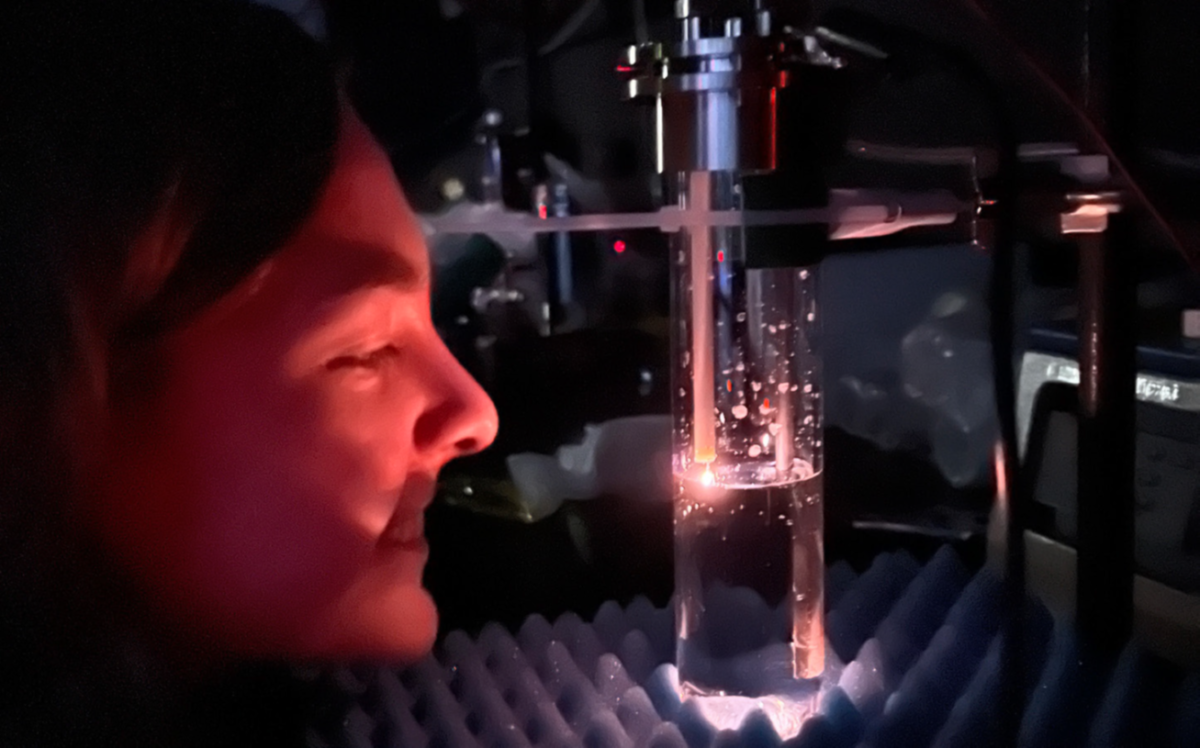
“We found a thousand ways not to make a molten salt spark gap,” Bryars said. “We think failures are very valuable.” The group said the path to the end-product was terribly challenging, but the trials influenced the research they are doing now.
The team’s final design featured flowing argon gas to protect the electrode and an optical fiber to retrieve the light emission, providing online material identification of molten salts using a glow discharge.
“Spectroscopy is another thing that makes us unique here,” Bryars said. A spectrometer will essentially read the different lights that come from the reactions within the molten salt when the team’s probe is in it. Every element has a different fingerprint, which reflects a different light, making it possible to identify which elements are in the molten salt.
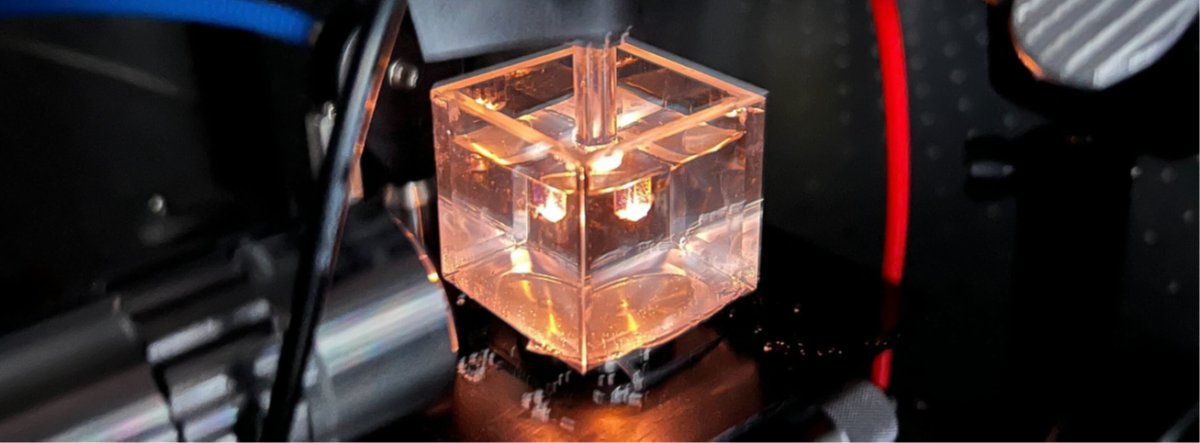
“The resulting spectrum is a plot of the intensity of the light to the light wavelength,” said Jugan. “You’ll see lots of spikes, and each of those spikes corresponds to a different wavelength and each of those wavelengths relates to a certain material or element. The more intense the spike, the higher presence of that light, or material.”
“The resulting spectrum is a plot of the intensity of the light to the light wavelength,” said Jugan. “You’ll see lots of spikes, and each of those spikes corresponds to a different wavelength and each of those wavelengths relates to a certain material or element. The more intense the spike, the higher presence of that light, or material.”
“If we use a spectrometer, it will tell us, ‘There is a spot here coming from potassium’ which has great advantages,” Bryars said.
The students’ hard work and research have made it possible to identify elements and materials in molten salt. This discovery can help monitor corrosion and fission products, identify uranium isotopes, and provide material quantification for molten salt reactors, which may facilitate commercialization and widespread use in the industry.
Next Steps
The team’s results show plenty of potential and the next steps are already in motion.
“We have a promising way to analyze these results,” Hahn said. “Now our focus is where we can improve and where we can put this.” The team is looking into commercialization and academic collaboration.
The group is bringing the product to national laboratories and other organizations in the nuclear energy industry for testing. Spurred on by their early success, the team isn’t finished with its research.
“I want to keep applying myself to these advanced reactors,” Bryars said. “I think there’s a lot of research that needs to be done and I hope nuclear engineering identifies problems that need to be solved. Wherever I can get the most work done is where I want to be.”